- Topological insulators can reduce transistor switching energy by a factor of four
- Defeating Boltzman’s tyranny, which puts a lower limit on operating voltage
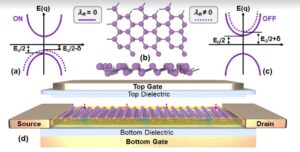
The theoretical study confirms a topological transistor would defeat Boltmann’s tyranny, a lower limit on operating energy
New FLEET research confirms the potential for topological materials to substantially reduce the energy consumed by computing.
The collaboration of FLEET researchers from University of Wollongong, Monash University and UNSW have shown in a theoretical study that using topological insulators rather than conventional semiconductors to make transistors could reduce the gate voltage by half, and the energy used by each transistor by a factor of four.
To accomplish this, they had to find a way to overcome the famous ‘Boltzmann’s tyranny’ that puts a lower limit on transistor switching energy.
They found a surprising result: gate voltage applied to a topological insulator could create a barrier to electron flow larger than the voltage itself times the electron charge, a result previously thought impossible.
The mission of the ARC Centre of Excellence in Future Low-Energy Electronics Technologies (FLEET) is to reduce the unsustainable energy load of information and computing technology (ICT), now consuming around 10% of global electricity.
Transistors: they’re not just in grandpa’s shed radio
Computer chips contain billions of transistors – tiny electrical switches that perform the basic switching operations of computing.
Individual transistors today are as small as 5 nanometres across (5 millionths of a millimetre).
Transistors use a voltage applied to a ‘gate’ electrode to switch on and off the current flowing between ‘source’ and ‘drain’ electrodes. The energy used to charge up the gate electrode is thrown away each time each transistor turns on and off. A typical computer has literally billions of transistors turning on and off billions of times each second, adding up to a lot of energy.
Conventional transistors are made from semiconductors, materials which possess a ‘bandgap’ or a range of energies within which electrons are forbidden. The action of the voltage applied to the gate is to move this range of forbidden energies to allow (the ‘on’ state) or block (the ‘off’ state) the energies at which incoming electrons are moving from source to drain.
In an ideal transistor, 1 volt applied to the gate would move up the range of energies blocked by 1 electron-volt.
Leakage ‘tyranny’ puts a lower limit on switching energy
How big a barrier is needed for the transistor to operate correctly?
The problem is that the energies of the electrons coming from the source are inherently ‘smeared out’ at finite temperature, so there are always a few electrons with sufficiently-high energy to make it over the barrier. This ‘leakage’ current leads to wasted energy.
Basic thermodynamic considerations require that to reduce the current by a factor of 10 requires raising the barrier by about 60 milli-electron-volts at room temperature. But to avoid wasted energy via leakage current requires the current to be reduced by a factor of about 100,000, or a barrier of about 300 milli-electron-volts, which requires a gate voltage of at least 300 milli-volts.
This minimum gate voltage puts a lower limit on switching energy.
This is called ‘Boltzmann’s tyranny’ after Ludwig Boltzmann who described the smearing of the energies of particles by temperature.
Boltzmann’s tyranny is thought to limit how small the operating gate voltage can be for a transistor, no matter what material it is made of.
Overcoming Boltzmann’s limit with new materials
Researchers in FLEET were curious whether a different effect could be used to make a barrier for electron flow in a transistor.
In some materials, an electric field can change the size of the bandgap. They wondered whether the electric field due to voltage applied to a gate electrode could be used to expand the bandgap and create a barrier to electrons. The answer is yes, but for typical materials this effect doesn’t beat Boltzmann’s tyranny: 1 volt applied to the gate can still only make a barrier no bigger than 1 electron-volt.
The researchers decided to look at a special class of materials called topological insulators, which have a bandgap that is effectively negative.
“Thin (two-dimensional) topological insulators are insulating in their interior, but conduct along their edges,” explains lead author Muhammad Nadeem (University of Wollongong). “In this state they can function as the ‘on’ state of a transistor, with current carried by the conducting edges.”
“The bandgap of a topological insulator can also be changed by an electric field,” says Nadeem. “When it becomes positive, the material is no longer a topological insulator, and no longer has conducting edges, acting much like a regular semiconductor, with the bandgap acting as a barrier to electron flow (the ‘off’ state).”
However, the research team found that, unlike a regular semiconductor, the increase in the bandgap (in electron-volts) in the topological insulator could be larger than the voltage applied to the gate (in volts), beating Boltzmann’s tyranny.
“The right topological materials could switch at voltages half as large as a similar conventional transistor, which would require only one fourth the energy,” says co-investigator Dimi Culcer (UNSW).
Where to from here?
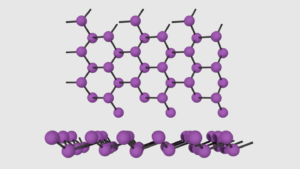
Bismuthene, a single atom-thick layer of bismuth atoms, is another candidate material for a topological transistor
Many challenges remain. The study is for the moment only theoretical. Co-investigator Xiaolin Wang (UOW) says “some of the candidate materials such as bismuthene, a single atom thick layer of bismuth arranged in a honeycomb structure, have only just begun to be studied in the laboratory, and haven’t yet been made into transistors.”
Other materials are still on the drawing board and it isn’t yet known how to synthesize them. “However,” says co-investigator Michael Fuhrer (Monash), “researchers within FLEET are working hard to make these new materials, characterize them, and incorporate them into electronic devices.”
The study
Overcoming Boltzmann’s Tyranny in a Transistor via the Topological Quantum Field Effect was published in Nano Letters in March 2021 (DOI 10.1021/acs.nanolett.1c00378)
The research was carried out by a collaboration between FLEET researchers at the University of Wollongong, University of New South Wales, and Monash University.
More information
- Contact Prof Michael Fuhrer (Monash University) michael.fuhrer@monash.edu
- Contact A/Prof Dimi Culcer (UNSW) d.culcer@unsw.edu.au
- Contact Prof Xiaolin Wang (University of Wollongong) xiaolin@uow.edu.au