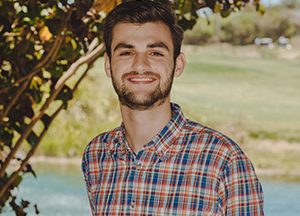
Oliver Stockdale (now at Kirchhoff-Institut für Physik, Universität Heidelberg) led the new vortex study while completing a MPhil at the University of Queensland
Physicists at the University of Queensland have shed light upon how tiny whirlpools (vortices) get stuck to obstacles in superfluids.
Superfluids are a quantum substance that can flow without viscosity and hence do not slow down due to friction.
A second defining feature of superfluids is that they only support quantised rotation – the vortices can only spin with strength one, two, or any integer amount. This means that, unlike in classical fluids, superfluid vortices persist indefinitely and do not lose their strength – they can, however, annihilate with other vortices spinning the other way.
Many properties of superfluidity – such as its ability to support dissipationless flow – strongly depend upon the dynamics of vortices. One recurring and common feature of the dynamics is the pinning and unpinning of vortices to defects in the superfluid. Yet, until now there has been no comprehensive description of these phenomena in zero temperature superfluids.
In this study, researchers from the ARC Centre of Excellence in Low-Energy Electronics Technologies (FLEET) at the University of Queensland reveal the fundamental mechanisms of vortex pinning and unpinning in a two-dimensional superfluid.
Once this enhanced theoretical knowledge of vortex pinning is confirmed in experiments in ultracold superfluid systems, extension to room-temperature superfluid systems could aid vortex control in future ultra-energy-efficient superfluid electronics.
“This problem is relevant to a hugely-different range of systems,” explains lead author Oliver Stockdale. “For instance, experiments with thin-film superfluid helium routinely encounter vortices interacting with defects caused by surface roughness at the nanoscale.”
“However, the very same problem is also relevant on astronomical scales. The sudden rotation glitches of neutron stars observed with radio telescopes are believed to be governed by the pinning and subsequent unpinning of upwards of 1019 vortices to the star’s crust.”
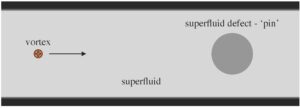
Figure 1. The simple test system designed by the UQ team modelled a single quantised vortex scattering off a circular defect
To study this complex phenomenon, the UQ authors designed the simplest system possible. They numerically simulated a single quantised vortex scattering off a circular superfluid defect and characterised the resulting dynamics. A simple schematic can be seen in Figure 1.
While the system itself is rather simple, the physics underpinning the processes are rather rich.
“We first developed an analytical model that describes the slowest dynamics of the vortex moving past the pinning site without scattering,” says co-author Matt Reeves. “We found the trajectories of a vortex past the defect strongly depend on its initial position. Some trajectories experience significant curvature as it passes the defect, while others don’t.”
In the regime when the vortex is moving very slowly, vortices never become pinned to the defect. This is known as the conservative regime. An example trajectory can be seen in Figure 2.
If, however, the vortex begins on a trajectory that exhibits significant curvature, the vortex can radiate energy in the form of sound. If it loses enough energy, it can fall onto a closed trajectory within the defect and becomes pinned. This regime, known as the ‘fall on’ regime, is also shown in Figure 2.
For both of these regimes, the vortex is moving relatively slowly. When the vortex approaches the defect at a faster rate, it can induce a vortex-antivortex pair within the defect. The approaching vortex annihilates with the newly created antivortex, leaving the newly created vortex to become pinned. Here, the energy loss comes in the form of the annihilation event through a burst of sound waves. This regime is known as the ‘pair creation’ regime and shown in Figure 3.
When the vortex is moving faster still, the same pair-creation process occurs. However, since the approaching vortex has more energy, the energy release via the sound burst is not enough to keep the newly created vortex on the defect. As a result, the new vortex escapes from the defect and no pinning occurs. Figure 3 shows a sample trajectory of this regime, known as the ‘too fast’ regime.
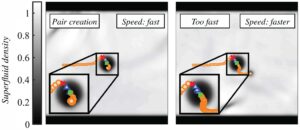
Figure 3. ‘Pair creation’ and ‘too fast’ regimes: fast approaching vortex ; new vortex escapes, with no pinning.
“Our analysis revealed that these four regimes characterise all the possible interactions and pinning mechanisms between superfluid vortices and defects,” explains co-author Matt Davis. “Each regime could be identified by considering the vortex energy and the emission of sound energy, which is crucial for vortex pinning.”
With the mechanisms of pinning now understood, the authors turned their focus to what type of defect best traps a vortex. By mapping out the phase diagram of vortex pinning as a function of vortex speed and initial position, they found that strong but small defects were most efficient at trapping vortices.
“With our enhanced knowledge of vortex pinning, we hope that our theoretical work can now be realised in experiments,” says Oliver. “We anticipate that ultracold atomic gas superfluids, where vortices and pinning defects can be routinely created and manipulated, would be an optimal setting to test our predictions. However, systems such as exciton-polariton condensates would be exciting to explore too due to their driven-dissipative nature and ability to host room-temperature superfluidity.”
An understanding of vortex dynamics in superfluid systems is essential for one of FLEET’s goals to develop ultra-efficient superfluid transistors. This study takes a step towards this goal by gaining a deeper understanding of how to control vortices in superfluids – a task that may be needed to mitigate adverse effects caused by vortices.
The paper Dynamical Mechanisms of Vortex Pinning in Superfluid Thin Films was published in Physical Review Letters in December 2021 (DOI: 10.1103/PhysRevLett.127.255302).
Videos of the corresponding trajectories shown in Figures 2 and 3 can be found here.