A team of Monash researchers have uncovered for the first time the full effects of interactions between exciton-polaritons and their associated dark excitonic reservoir.
Exciton-polaritons (‘polaritons’, for short) are hybrid mixtures of light and matter that inherit the best properties of both. They form in semiconductors sandwiched between two mirrors, through which a laser is shone.
“Polaritons capture useful properties of both matter and light”, says lead author Kenneth Choo. “Their ‘matter’ part means we can manipulate them through their interactions. Meanwhile, the ‘light’ part makes the polariton almost massless, resulting in the formation of a superfluid condensate that flows effortlessly.
“Put them together, and a new kind of ‘liquid light’ that we can guide and control is formed, potentially the basis for the next generation of computing that uses light to build circuits,” says Kenneth, who is a PhD candidate at Monash.
But with every yang there is a yin, and so it is with the polariton’s dark counterpart – a dark exciton.
These dark excitons are uncoupled to light yet still able to interact with the polaritons.
“Whenever the polaritons are formed in experiments, they tend to be accompanied by a substantial reservoir of these dark excitons which form together with them. The fact that they are dark, however, means it has been difficult to see their true effect,” says corresponding author Prof Meera Parish.
A famous technique dresses up for the occasion
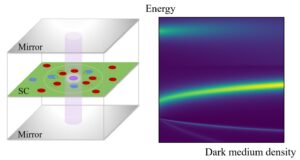
Left: The device comprises a 2D semiconductor between two mirrors, the polariton in purple, and the dark excitons in red or blue depending on spin. The effect of dark excitons can be encapsulated in the polaron, the white dashed circle. Right: The resulting transmission spectrum.
The Monash researchers used a framework known as Fermi liquid theory to explain the effect of the dark reservoir on the bright polaritons. The essential idea is to map the (many, many!) possible interactions onto a new ‘quasiparticle’ – one with different masses, energies and lifetimes to the original. This allows the many-particle system to be treated as if it were a single particle.
One such quasiparticle is the polaron, which can be thought of as a ‘zone of influence’ around the original particle.
By using this technique and including all possible two-particle interactions, they found that the bright polariton becomes ‘dressed’ by excitations of the dark medium, forming a new quasiparticle called the polaron polariton.
Physically, this shows up as a reduction in the strength of the light-matter coupling as the dark exciton density is increased, an effect which is commonly seen in experiment.
In contrast, the strength of the interactions between the dark reservoir and the polaritons are increased, especially when the spins of the reservoir and polariton are different. This effect can be so strong that an additional quasiparticle is generated – the biexciton polariton – which is associated with the bound state of two opposite-spin excitons. This phenomenon, known as a Feshbach resonance, was first observed in ultracold atomic gases, and offers the promise of being able to tune the interaction strength to whatever value is desired. It would then be possible to create reservoir ‘hills’ and ‘valleys’ that guide the flow of the polariton condensate, and then change them around at the flick of a (laser) switch.
What’s next?
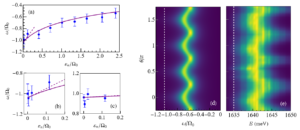
Experimental confirmation of the theory. Left: Observed energies (blue dots) align with theoretical prediction (purple lines). Right: Observed spin variation, compared with theoretical prediction.
This study opens up new opportunities in the field of reservoir engineering. Already, there has been experimental progress in using this dark reservoir to trap and focus the bright polaritons, and the study shows how the reservoir can be used to fundamentally change the polaritons themselves.
In addition, this study provides a new way to look at old results – by accounting for the hitherto unknown influence of the reservoir, a more accurate determination of interaction strengths can be obtained.
The study
The study ‘Polaronic polariton quasiparticles in a dark excitonic medium’ was published in Physical Review B as an Editor’s Suggestion in May 2024 (DOI 10.1103/PhysRevB.109.195432).
This study uses experimental data obtained at the University of Grenoble Alpes. The work was supported by the ARC Centre of Excellence in Future Low-Energy Electronics Technologies (FLEET), ARC Future Fellowship Grants, and an ARC Research Training Program scholarship.
More information
The Theory of Quantum Matter Group at Monash is led by Prof Meera Parish, and studies strong many-body correlations in a variety of quantum systems, such as 2D semiconductors and ultracold atomic gases.
The work done in this particular study falls under FLEET Themes 2 and 3.
- Kenneth Choo kenneth.choo@monash.edu
- Prof Meera Parish meera.parish@monash.edu