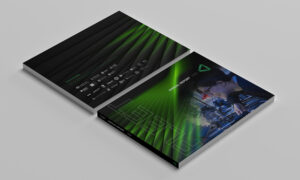
This is an extract from the 2022 FLEET annual report [read the full report online] See previous section (Research Translation) / see next (Collaboration)
The above approaches are enabled by the following technologies:
FLEET’s approach is multidisciplinary, combining efforts across condensed-matter, cold-atom physics, material science and nanofabrication.
FLEET will:
- Develop and progress new concepts for low-energy electronics
- Increase the global science community’s fundamental understanding of quantum materials and electronic devices
- Build Australian research capacity for quantum materials, semiconductors and electronic devices
- Enable discoveries at the scientific frontier.
Research theme 1: Topological materials
FLEET’s topological materials research theme seeks to achieve electrical current flow with near-zero resistance, based on a paradigm shift in the understanding of condensed-matter physics and materials science: the advent of topological insulators.
Unlike conventional insulators, which do not conduct electricity at all, topological insulators conduct electricity, but only along their edges.
Along those topological edge paths, electrons can only move in one direction, without the ‘backscattering’ that dissipates energy in conventional electronics.
FLEET’s challenge is to create topological materials that will operate as insulators in their interior and have switchable conduction paths along their edges.
Topological transistors will ‘switch’, just as a traditional (silicon-based) CMOS transistor does, with a ‘controlling’ voltage switching the edge paths between being a topological insulator (‘on’) and a conventional insulator (‘off’).
For the new technology to become a viable alternative to traditional transistors, the desired properties must be achievable at room temperature (otherwise, more energy is lost in maintaining ultra-low temperatures than is saved by the low-energy switching).
Approaches used are:
- Magnetic topological insulators and quantum anomalous Hall effect (QAHE)
- Topological Dirac semimetals
- Artificial topological systems.
2022 THEME 1 HIGHLIGHTS
- Optimising low-energy topological switching of 2D Xene nanoribbons (see case study)
- Forming self-assembled kagome lattices using metal-organic frameworks, with lattice-electron interactions creating novel electronic states towards a low-power ‘Mott’ transistor
- Theoretically proving topological materials exhibit novel, non-linear electrical and optical properties usable in material characterisation and new device applications
- Achieving novel functionalities and improved properties in piezoelectric materials using carefully controlled crystal growth.
IN 2023 FLEET WILL:
- Build on world-leading techniques FLEET has developed for fabricating nanoscale artificial lattices using both ‘bottom-up’ self assembly and top-down lithography approaches to study devices with designer quantum properties
- Exploit the flexibility of van-der Waals systems to combine materials with very different electronic and magnetic properties, enhancing the properties of topological insulators towards a functional topological transistor
- Develop theoretical models to quantitatively understand effects of spin-orbit interaction on electronic properties of nanoscale semiconductor devices, and the interplay of magnetism and topology in topological materials.
RESEARCH THEME 2: Exciton superfluids
FLEET’s second research theme uses a quantum state known as a superfluid to achieve electrical current flow with minimal wasted dissipation of energy.
In a superfluid, scattering is prohibited by quantum statistics, so electrical current can flow without resistance.
A superfluid is a quantum state in which all particles flow with the same momentum, and no energy is lost to other motion. Particles and quasiparticles, including both excitons and exciton-polaritons, can form a superfluid.
Researchers are seeking to create superfluid flows using three approaches:
- Exciton-polariton bosonic condensation in atomically-thin materials
- Topologically-protected exciton-polariton flow
- Exciton superfluids in twin-layer materials.
If exciton-superfluid devices are to be a viable, low-energy alternative to conventional electronic devices, they must be able to operate at room temperature, without energy-intensive cooling.
Thus, FLEET seeks to achieve superfluid flow at room temperature, using atomically-thin semiconductors as the medium for the superfluid.
2022 Theme 2 HIGHLIGHTS
- Investigating, experimentally and theoretically, interactions of Fermi polarons in a charge-doped monolayer WS2 (see case study)
- Realising a low-temperature p-type Ohmic contact for electrical transport studies in monolayer WS2
- Observing enhancement of coherence and ground state population of room-temperature WS2 polaritons in an engineered trap
- Developing a scalable method of integrating monolayer WS2 into polymer-spaced optical microcavities for strong light–matter coupling
- Directly measuring WS2 biexcitons by multidimensional coherent spectroscopy.
IN 2023 FLEET WILL:
- Continue working towards superfluid condensation of exciton-polaritons in TMD monolayers at room temperature
- Study multi-body complexes and long-lived dark excitons in TMD monolayers, and explore their potential for superfluid condensation
- Continue to investigate the transition to BCS regime in exciton-polariton systems
- Theoretically explore the behaviour of dissipative superfluids in channels.
THEME 3: Light-transformed materials
The zero-resistance paths for electrical current sought at FLEET can be created using two non-equilibrium mechanisms:
- Short (femtosecond), intense bursts of light temporarily forcing matter to adopt a new, distinct topological state
- Dynamically-engineered dissipationless transport.
Very short, intense pulses of light are used to force materials to become topological insulators (see Research theme 1) or to shift into a superfluid state (see Research theme 2).
The forced state achieved is only temporary, but researchers learn an enormous amount about the fundamental physics of topological insulators and superfluids as they observe the material shifting between natural and forced states over a period of several microseconds.
By using ultra-short pulses to switch between the dissipationless-conducting and normal states, we can also create ultra-fast opto-electronic switching of this dissipationless current.
The second approach typically uses periodic perturbations (usually optical) to modify the time-averaged behaviour of the system.
2022 Theme 3 HIGHLIGHTS
- Measuring Higgs mode oscillations in a superfluid gas of fermions (see case study)
- Electrostatically controlling broadband terahertz complex conductivity of graphene
- Quantum virial expansion of exciton-polarons
- Experimentally studying polaron–polaron interactions in monolayer WS2
- Theoretically observing bistability in a driven-dissipative superfluid
- Developing a theory of thermalisation of tunnel-coupled nonequilibrium condensates.
IN 2023 FLEET WILL:
- Demonstrate Floquet control of band structure in graphene
- Continue to develop approaches to identify topological states in Floquet system using terahertz spectroscopy
- Develop quantitative probes for impurities and non-equilibrium dynamics in a 2D Fermi gas
- Investigate quantum kicked-rotor (Floquet) system with spin-orbit coupling
- Demonstrate coherent quantum microscopy probe to image currents in 2D materials
- Further develop theory of driven-dissipative (nonequilibrium) transport of a superfluid.
TECHNOLOGY A: Atomically-thin materials
Each of FLEET’s three research themes is significantly enabled by the science of novel, atomically-thin, two-dimensional (2D) materials.
These are materials that can be as thin as just one single layer of atoms, with resulting unusual and
useful electronic properties.
To provide these materials, from bulk crystals to thin films to atomically-thin layers, FLEET draws on extensive expertise in materials synthesis in Australia and internationally.
The most well-known atomically-thin material is graphene, a 2D sheet of carbon atoms that is an extraordinarily-good electrical conductor.
FLEET scientists use other atomically-thin materials in their search for materials possessing the necessary properties for topological and exciton-superfluid states.
2022 HIGHLIGHTS
- Constructing a large-bandgap quantum anomalous Hall insulator heterostructure (see case study)
- Observing giant, negative magnetoresistance in titanium-doped 2D ferromagnetic Cr2Se3
- Investigating magneto-transport towards tuning Berry phase in magnetic-doped 3D topological insulator BI2Se3
- Confirming weak antilocalisation in topological semimetal Ta0.7Nb0.3Sb2 single crystals
- Possible permanent Dirac-to Weyl-semimetal phase transition by ion implantation
- Designing bismuth ferrite multiferroic films with giant piezoelectric properties
- Understanding the role of defects in LaNiO3 rare-earth nickelate films
- Creating topological solitons in bismuth ferrite superlattices
- Developing a new scanning probe technique (tomographic atomic force microscopy) to study topological defects under the surface of a ferroelectric film.
IN 2023 FLEET WILL:
- Develop resonant tunnelling heterostructures in bismuth ferrite with engineered topological defects
- Develop low-energy switching routes in bismuth ferrite films
- Develop new high-entropy oxide spintronic materials.
TECHNOLOGY B: Nanodevice fabrication
FLEET’s research sits at the very boundary of what is possible in condensed-matter physics. Thus, nanoscale fabrication of functioning devices will be key to the Centre’s ultimate success.
Specialised techniques are needed to integrate novel atomically-thin, two-dimensional (2D) materials into high-quality, high-performance nanodevices.
For example, successful development of functional topological transistors will require atomically-thin topological insulators to be integrated with electrical gates. And exciton-polariton condensate devices will require atomically-thin semiconductors to be integrated with optical cavities.
Nanodevice fabrication and characterisation link many of FLEET’s groups and nodes. Some groups bring expertise in device fabrication, while other groups are stronger in device characterisation.
FLEET brings Australian strength in microfabrication and nanofabrication together with world-leading expertise in van der Waals (vdW) hetero-structure fabrication to build the capacity for advanced atomically-thin device fabrication.
2022 Technology B HIGHLIGHTS
- Manipulating interlayer magnetic coupling in van-der Waals hetero-structures (see case study)
- Realising room-temperature, electrically-tuned magnetic phase transition in vdW materials
- Achieving resonant tunnelling in ferroelectric heterostructures, including polarisation-mediated negative differential resistance
- Strategy for single-gate operation of a topological quantum field-effect transistor to beat Boltzmann’s tyranny
- Showing that proximity coupling to an antiferromagnet can simultaneously beat Boltzmann’s tyranny and preserve the dissipationless “ON” state
- Demonstrating Ga2O3 glass as a protective layer for graphene which reduces the temperature-dependent resistivity.
IN 2023 FLEET WILL:
- Work towards realising room-temperature magnetic proximity effect in vdW hetero-structures
- Work towards realising room-temperature spin-transport devices and spin-torque devices based on vdW hetero-structures.