First year physics students enthusiastic to explore ‘new’ physics
Measuring quantum Hall effect with liquid helium-3, a Cold War side-product
Window to topological materials and future electronics
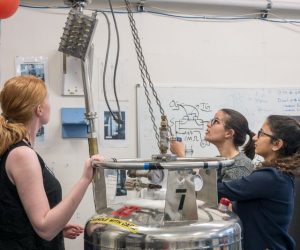
Karina Hudson (left) directs first year students Greta Paget (centre) and Keshavi Charde (right) in the lab experiment
UNSW first year physics students have been measuring the quantum Hall effect (QHE), a relatively new piece of physics recognised by the 1985 Nobel Prize in Physics, which requires precision experimental setup.
When first-year physics students Olivia Kong and Ian Thorvaldson discovered that the QHE they had learned about in their Introductory Skills for Science course could be measured in the labs at UNSW, they wanted in.
“It’s easy to just accept the results of experiments you learn in lectures, memorise them for an exam and forget about them,” says Ian. “But actually doing the experiment gave so much more meaning to the effect than we could have got from lectures.”
The experiments have now been expanded to a wider group of undergraduates under the watchful supervision of Karina Hudson, a UNSW Physics PhD student.
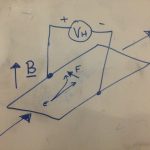
Hall voltage, perpendicular to longitudinal current, the result of an applied magnetic field forcing electrons towards one side
The quantum Hall effect (QHE) is a quantum-mechanical version of the Hall effect, in which a small voltage difference is created perpendicular to a current flow by an applied magnetic field.
The quantum Hall effect is observed in two-dimensional systems at low temperatures within very strong magnetic fields, in which the Hall resistance undergoes quantum transitions — ie, it varies in discrete steps rather than smoothly, as shown below.
In the experiments, students must use helium-3 to cool ultra-high quality gallium-arsenide semiconductor chips to -272.9°C (only 0.25 degrees above absolute zero). Helium-3 is an extremely rare isotope, with most of the world’s supplies historically being siphoned off as a by-product of radioactive decay in nuclear warheads.
A powerful superconducting magnet is used to create the large magnetic field (up to 2 Tesla) required to induce the quantum Hall effect.
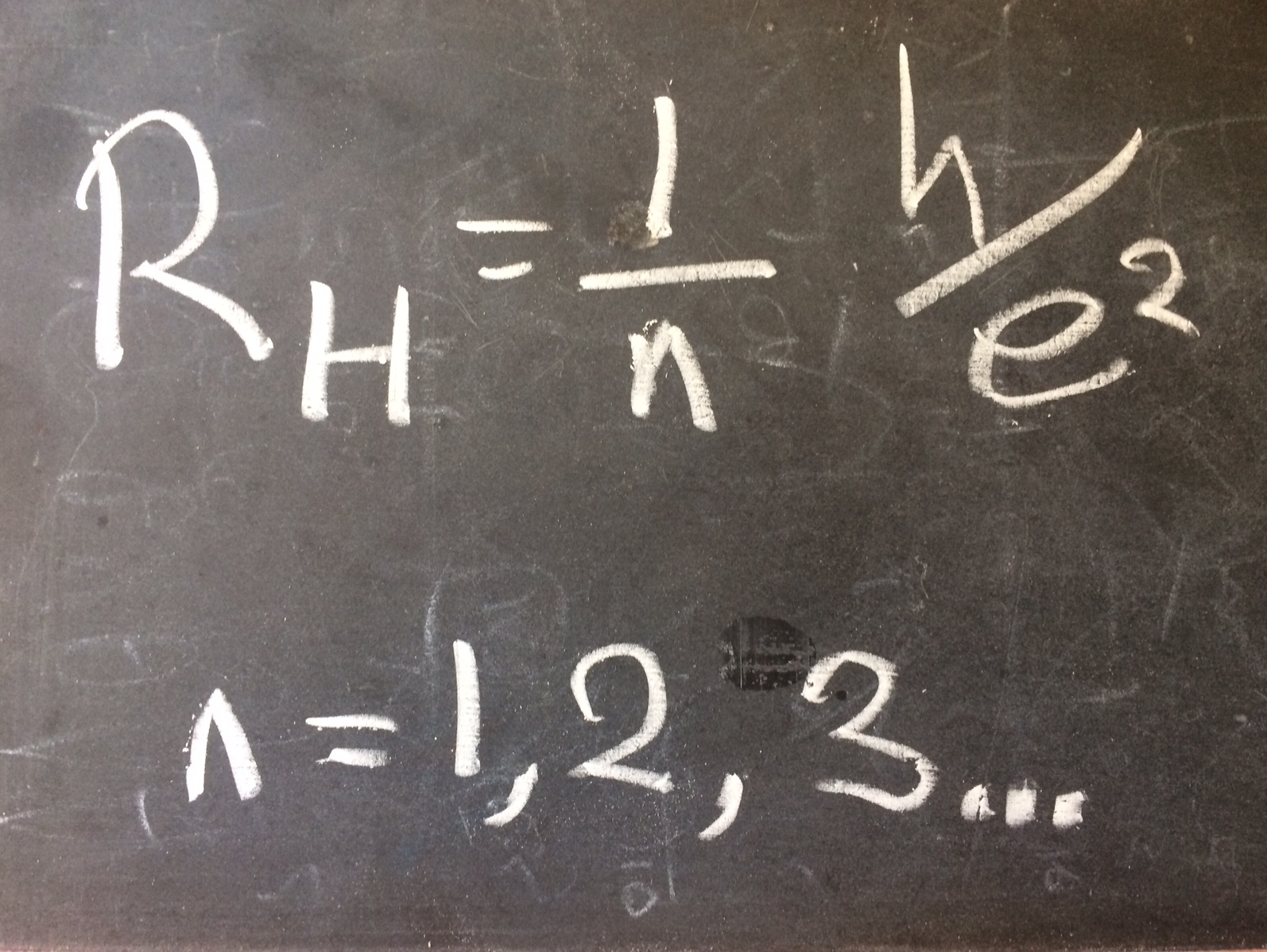
The equation governing QHE, with Hall resistance varying in integral steps proportional to the Klitzing constant, h/e2
The semiconductor chips contain a thin (two-dimensional) sheet of highly mobile electrons, and at these low temperatures the students confirmed that the Hall resistance develops flat plateaus, where the Hall resistance is independent of the magnetic field. At these plateaus the Hall resistance depends only on the electron charge and Planck’s constant, while the longitudinal resistivity drops to zero.
Students discovered the challenges of ultra-cold, ultra-precise experimentation, including:
- Loading the 3He fridge correctly to avoid air gaps – any air left in the fridge will freeze solid at these temperatures, and block the lines
- Correct electrical grounding, allowing measurement of currents as small as 10 billionths of an Amp
- Analysis to reproduce the famous quantum Hall effect plots (shown).
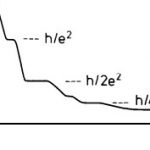
Quantised Hall resistance, measured by Klitzing in 1980 – awarded the 1985 Nobel Prize in Physics for the discovery
Ian and Olivia’s report on the experiment has been used by the next pair of QHE students, Keshavi Charde and Greta Paget.
As well as learning their way around sophisticated experimental apparatus, students have been able to recreate extremely important quantum physics experiments themselves, rather than just reading about it in a textbook.
“It’s awesome to be able to do the Quantum Hall Effect in the lab,” says Ian. “It’s one thing to study the theory in class, but another thing entirely to see it actually works in the real world.”
“I also like how we got to see the procedures and engineering behind the experiment – all the complicated setup needed to do an experiment at ultra-cold temperatures, only a few tenths of a degree above absolute zero. I was thrilled and a little surprised that they let first year’s play with all their delicate equipment!”
Experiments conducted under extreme conditions can sound almost ho-hum in the lecture theatre, but it’s very different when you have to do it yourself…
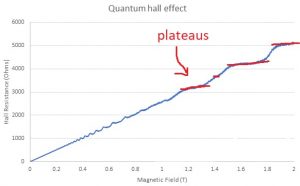
“When we saw our data plateauing at the expected areas, we were almost in disbelief – our experiment was working! The gap was bridged between learning theory in the classroom and collecting our own data.”
—Olivia Kong, UNSW student
“We learn about physics at the extremities of the universe – calculations near the speed of light or temperatures at a fraction of a Kelvin…” says Olivia Kong. “When you get the chance to actually perform these experiments, it’s a reality check: these numbers and theories that we’ve calculated in class are well tested through detailed experiments.”
“It’s been a real pleasure to have such bright and enthusiastic students in the labs,” says supervisor Karina Hudson. “They got a real buzz out of reproducing Nobel prize-winning results for themselves, and learning how to operate precision experimental equipment.”
Karina’s own research project studies how spin-orbit interaction in 2D systems affects charged particles in one-dimensional quantum wires.
Topology and future electronics research at UNSW
Karina’s supervisor Prof Alex Hamilton, and several of their colleagues, work with a ‘successor’ to QHE.
These researchers utilise a new class of materials known as topological insulators to create electronic pathways in which current can flow without any wasted dissipation of energy. It is cutting-edge physics. The importance of topological insulators were recognised by the 2016 Nobel Prize in Physics.
The dissipationless edge states of topological materials depend on a ‘successor’ to QHE, the quantum anomalous Hall effect (QAHE), referred to as ‘anomalous’ because it occurs in the absence of any applied magnetic field. In topological materials, the sideways force is provided by spin-orbit interaction, an interaction between electrons’ movement and their inherent magnetic moment.
Alex and colleagues conduct the research as members of FLEET, an Australian Research Council-funded centre that is using topological materials to develop a new generation of ultra-low energy electronics.
FLEET (the ARC Centre of Excellence in Future Low-Energy Electronics Technologies) is driven by need to reduce the energy used in informational technology and computation, which already represents 5% of global electricity.
Alex Hamilton works with five other FLEET Chief Investigators at UNSW: Dimi Culcer, Oleh Klochan, Jan Seidel, Oleg Sushkov and Nagarajan Valanoor and over 20 other researchers from the UNSW School of Physics and the UNSW School of Materials Science and Engineering.
For more information:
- Prof Alex Hamilton alex.hamilton@unsw.edu.au
- Isabelle Dubach UNSW Science Media and Content Manager i.dubach@unsw.edu.au
- FLEET UNSW website unsw.FLEET.org.au
- Social media @FLEETCentre
- Errol Hunt FLEET communications coordinator, media@fleet.org.au
The physics: how it works
A classical explanation of the quantised Hall effect (QHE). With thanks to Olivia Kong for the animations and Charles Kane for the electron circles diagram.
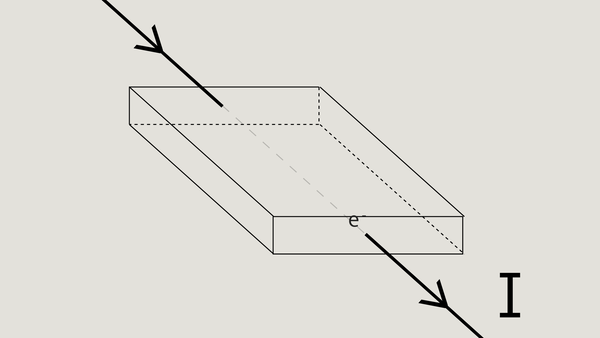
Fig 1. The normal flow of electrons in a conducting material
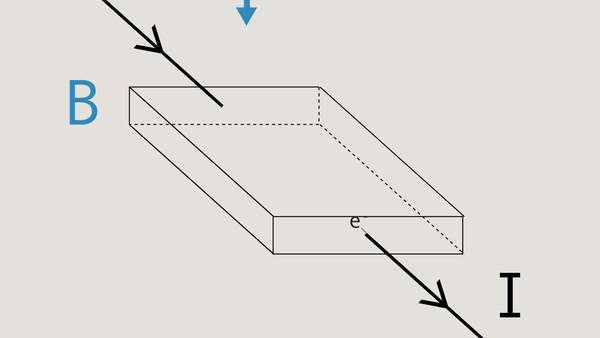
Fig 2 An applied magnetic field (B) diverts electrons to one side (this is called the Lorentz force, and is the same force as powers every electric motor: simply put, a magnetic field acting on a charged particle causes a force to the side). This forced diversion of electrical charge to one side causes a small voltage difference across the width of the material, perpendicular to the current flow, which is known as the Hall voltage.
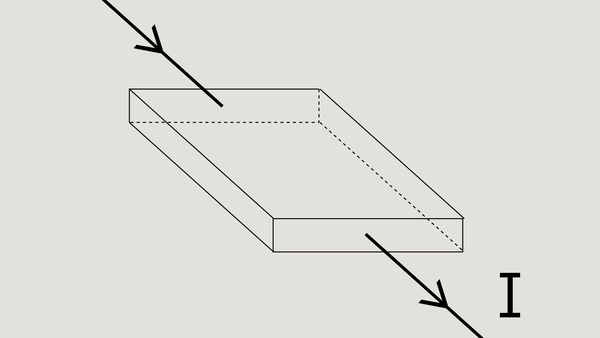
Fig 3 In a 2D material, a very strong magnetic field (B) forces electrons to move in circles, so there is no effective current flow through the material – thus it is an insulator in its centre.
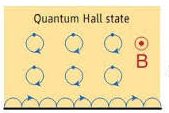
Fig 4 Electrons forced into tight circles in the interior of the material make that area an electrical insulator (ie, there no net movement of charge). The only possible movement is by ‘half circles’ along it’s edges, which is where the current is carried.
The movement of electrons constrained to two dimensions is dictated by their wave nature. These electrons moving in circles form standing waves that can only be an integer multiple of the electron’s de Broglie wavelength – this is where the quantisation comes from in the quantised Hall effect.