- Molybdenum based compounds could provide key to hydrogen production for future zero-emissions energy
- RMIT/Monash collaboration opens promising route towards alkaline hydrogen production
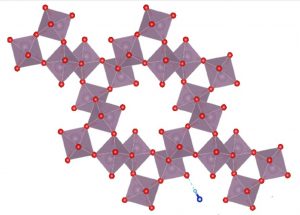
Crystal structure of molybdenum-trioxide (MoO3) doped with NH4+ ions, with regular orthorhombic (rectangular) structure altered to hexagonal
A FLEET study combining experimental expertise at RMIT with theoretical modelling at Monash University opens a new route towards efficient, cost-effective production of hydrogen.
Researchers discovered that ammonium-doped, hexagonal molybdenum oxide (MoO3) displays extremely promising electronic and material properties for use as a catalyst in production of hydrogen from water.
The resulting, improved electrochemical activity and exceptional stability confirm promise for this technique in future hydrogen production.
Molybdenum catalysts and hydrogen power
Hydrogen gas is a highly attractive alternative fuel, being carbon-neutral, free of environmentally damaging bi-products, and fully recyclable.
The technology’s major challenge is the sustainable and efficient production of hydrogen gas.
Room-temperature, alkaline water electrolysis is one of the most-promising hydrogen production technologies, despite the high energy requirements of the alkaline process (conversely, an acidic process has higher costs, but uses less energy).
And so the search is on for an economical catalyst with low energy-requirements, and which is resistant to corrosion in an alkaline environment.
Molybdenum based compounds are an emerging class of catalytic materials for hydrogen production in an acidic electrolyte, but most of these compounds lose catalytic performance and exhibit poor long-term stability in alkaline media.
The chemically-inert Molybdenum trioxide (MoO3) can be can be made more conductive via the introduction of oxygen vacancies, which also favours the adsorption of water molecules, hence lowering the energy required to separate out hydrogen.
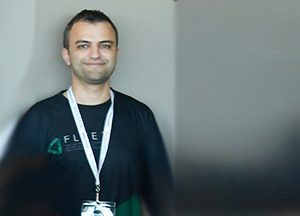
“Atomic force microscopy (AFM) and X-ray diffraction (XRD) were used to assess the material’s structure and hydrogen evolution reaction rate at the labs at RMIT”—Studied by PhD candidate Farjana Haque and FLEET Research Fellow Dr Ali Zavabeti (both at RMIT University)
The key was to induce crystal phase transition via doping the material with ammonium ions. This alters the regular orthorhombic (rectangular) structure to a hexagonal crystal, which drastically improves stability.
This hexagonal crystal structure causes formation of tunnel-like pores in the material, which assist with stability, and also improve energy-performance, by increasing available reaction area and lowering hydrogen adsorption energy.
Electrons injected during the doping process also improve electron conductivity.
Measured catalytic activity was higher than any other, previously-reported Molybdenum compounds, with an exceptional stability exceeding 40 hours of operation.
The researchers demonstrated that the formation of highly-ordered intra-crystalline pores of 2D molybdenum oxide enables efficient and extremely stable hydrogen evolution reaction (HER) activity in an alkaline medium.
Compared to similar studies, the researchers’ molybdenum catalyst demonstrated significantly-enhanced HER activity, confirming that the doping-driven formation of highly-ordered intra-crystalline pores on 2D materials could be a feasible design strategy for high-performance, non-metal catalysts for alkaline hydrogen evolution.
The study
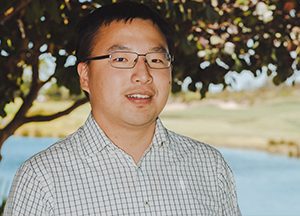
“Theoretical calculations identified active atomic sites for hydrogen adsorption of hydrogen on MoO3, revealing the physical origin of the stabilised catalytic activity”—FLEET Research Fellow Dr Yuefeng Yin (Monash University)
‘Ordered intracrystalline pores in planar molybdenum oxide for enhanced alkaline hydrogen evolution’ was published in the Journal of Materials Chemistry A in January this year. (DOI 10.1039/C8TA08330D)
As well as funding by the Australian Research Council, the study benefited from the facilities and expertise of the RMIT MicroNano Research Facility (MNRF), the RMIT Microscopy and Microanalysis Facility (RMMF) and Frank Antolasic, Applied Chemistry.
Support was also offered by Hainan Province’s Finance Science and technology project and China’s National Natural Science Foundation.
Theoretical models were run using computational facilities at the Monash Campus Cluster, NCI National Facility (ANU) and the Pawsey Supercomputing Facility. Anthony Chesman (CSIRO Manufacturing, Ian Wark Laboratories) performed PESA experiments.
Novel materials at FLEET
The properties of novel materials are studied at FLEET, an Australian Research Council Centre of Excellence, within the Centre’s Enabling technology A.
The Centre for Future Low-Energy Electronics Technologies (FLEET) is a collaboration of over a hundred researchers, seeking to develop ultra-low energy electronics to face the challenge of energy use in computation, which already consumes 8% of global electricity, and is doubling each decade.
More information
- Contact Kourosh Kalantar-zadeh (experiment) k.kalantar-zadeh@unsw.edu.au (RMIT/UNSW)
- Contact Nikhil Medhekar (theory) nikhil.medhekar@monash.edu (Monash)
- Connect at @FLEETCentre
- Read FLEET Enabling technology A: novel materials news