What can you do with simple shapes? Create a type of animation that will surprise you.
It is cool quantum art, yet our understanding of moiré patterns is behind the development of novel materials to enable quantum computing, and to develop energy-efficient digital technologies, data storage and information and electricity transmission. It all comes down to magic angles and what is called twistronics. FLEET is one of the research groups around the world working in this area of research. And no, twistronics is not the latest music genre or a LEGO® megabot.
What you need:
- two printouts of attached shapes, one on regular paper and one on a transparency
- drawing pin
What to do:
- Print out two copies of the squares, triangles or hexagons image from the links here. One should be on regular paper, the other on a transparency. You can print on transparencies at places like Officeworks if you don’t have your own printer.
- Line up the two printouts so they are directly over one another, with the transparency on top. Use a drawing pin to push through the exact centre (there is a small dot to identify the centre location). It is best to push the pin through the back of the sheets.
- Slowly rotate the transparent sheet. See what shapes appear.
What happens:
You will get different shapes appearing and certain movement through the images. This is known as a Moiré pattern, where patterns appear through the transparent gaps on the top sheet.
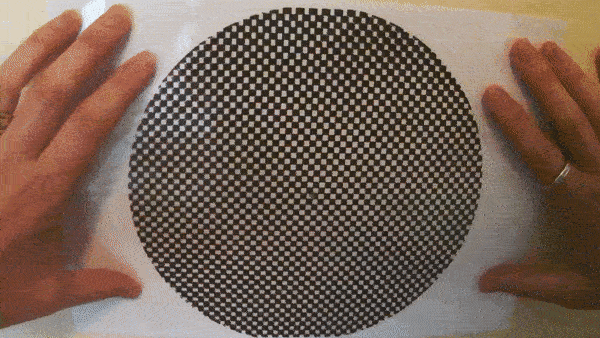
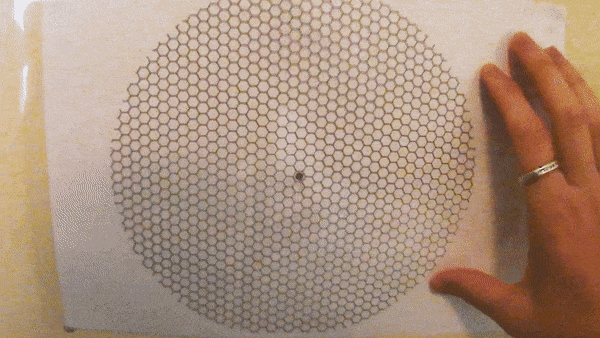
Moiré patterns in modern research
Scientists use moiré patterns at the atomic level to create novel materials, an area of research that FLEET is heavily involved in. This new research direction exploits recently discovered two-dimensional materials – crystalline solid sheets of atoms that can be just one to a few atoms thick.
In these two-dimensional materials, as in many solids (called crystals), the atoms are ordered and form an array (like the arrays made by the black shapes in the sheets of paper used in the above experiment). And just like you did with the moiré patterns on the sheets of paper in the experiment, by placing one of these 2D crystal arrays on top of the other and changing the angle of one of the layers – ie twisting it slightly to that ‘magic angle’ – scientists can create new hybrid materials with novel electronic properties such as changing the material from a conducting metal to an insulator, or even achieving superconductivity. That is, it is that slight yet precise twist of one of the arrays that enables the change in the electrical or mechanical properties of the material. Hence the term, ‘Twistronics’.
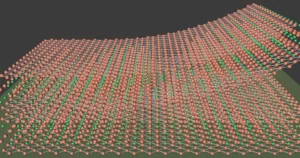
A material made at Princeton has highest electron mobility among known layered magnetic materials. Electrons inside the material, gadolinium tritelluride, are able to travel at high speeds with minimal scattering, reducing the heat dissipation of any electronic devices built from it. Credit: Shiming Lei
From – https://scitechdaily.com/new-layered-magnetic-material-has-properties-useful-for-twistronic-devices-and-spintronics/
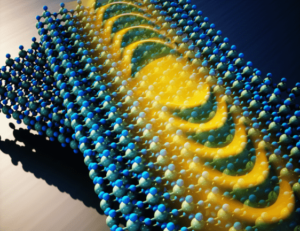
Twisted photonics: A bilayer of molybdenum trioxide supports highly unusual light propagation along straight paths when the two layers are rotated with respect to each other at the photonic magic angle. (Courtesy: Advanced Science Research Center, CUNY)
Several experimental research teams have now recently succeeded in controlling or precisely tuning the angle between the two layers to create novel “moiré materials”. Before this, it was lots of trial and error to achieve that ‘magic angle’. This control is key to advancing the field of Twistronics and some researchers consider that understanding moiré patterns is key to understanding and achieving room temperature superconductivity.
What is superconductivity: Superconductivity is a quantum state where an electric current will circulate indefinitely without resistance. That is, the current will circulate with zero energy loss. Achieving room temperature superconductivity is one of the holy grails of science/technology because it could be used in everyday applications and potentially contribute to the reduction in our global energy consumption. At the moment superconductivity can only be achieved at really cold temperatures (about -170 degrees Celsius for ‘high’ temperature superconductors).
Learn more about how Moire effects are being used in quantum physics
What FLEET is up to in the field of Twistronics
Explainer: Moire patterns in graphene and plastic
Applying ‘magic angle’ twistronics to manipulate the flow of light
Overview from around the world
Twistronics’ tunes 2D material properties
Applying ‘magic angle’ twistronics to manipulate the flow of light
With a Simple Twist, a ‘Magic’ Material Is Now the Big Thing in Physics
New Layered Magnetic Material Has Properties Useful for Twistronic Devices and Spintronics
Post by Olivier Bleu (FLEET Research Fellow)
Back to Home Science activities.