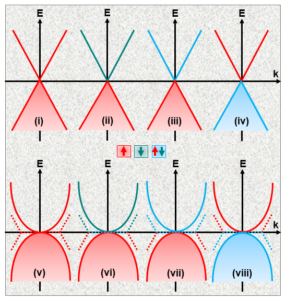
Energy-momentum dispersion of Dirac spin gapless semiconductors (i-iv) and parabolic spin gapless semiconductors (v-viii). Dashed bands represent flat-band spin gapless semiconductors.
FLEET researchers from the University of Wollongong have revisited the concept of spin gapless semiconductors (SGSs), one of the most fascinating materials classes in quantum condensed-matter physics, originally proposed by Xiaolin Wang in 2008.
In SGSs, low energy bands constitute a spin gapless energy-momentum dispersion. I.e., gapless spectrum in one of the spin sectors, while a gapped spectrum in the other.
In light of this, SGSs act like a bridge between magnetic half-metals and magnetic semiconductors. The spin gapless dispersion could be linear or parabolic and usually categorized as Dirac SGSs and parabolic SGSs, that are further classified in four different types with distinct spin texture of valence and conduction bands.
Quantum materials
Quantum materials are solid state systems characterised by non-trivial quantum mechanical effects on the microscopic electronic states that give rise to exotic properties at the macroscopic scale.
For instance, entanglement and topology of the electron wavefunction in quantum materials lead to a rich variety of quantum states/phases that do not exist in conventional materials.
The electron wavefunction in conventional materials is also characterised by quantum mechanics, but in a trivial way such that the conventional states/phases can often be described using classical physics.
In quantum materials, the quantum states/phases, which are less classically describable than conventional states/phases, are distinguished by the existence of quantum phase transitions.
In topological quantum materials, topology of electronic wavefunctions in the bulk (i.e., the interior of the material) induces metallic states at the boundary, known as bulk-boundary correspondence.
Among several non-trivial mechanisms, one of the key roles is played by a relativistic quantum phenomenon called spin-orbit interaction (SOI).
In topological insulators, SOI opens an energy gap and induces a correspondence between microscopic bulk and macroscopic boundary states. In topological semimetals, bulk states may remain protected against SOI, but the interaction can transform nodal-line semimetals to Dirac and Weyl semimetals.
In either case, SOI determines the spin texture of electronics states, both in the bulk and on the boundaries, and is directly linked to several other non-trivial quantum mechanical effects such as Berry curvature, spin-orbit torque, and chirality of charge and spin transport.
Spin gapless quantum materials
Quantum materials that exhibit spin gapless behaviour in spin or pseudospin space are referred to as spin gapless quantum materials (SGQMs). That is, along with magnetic topological insulators, topological nodal-line spin gapless semimetals and non-trivial Rashba materials, SGSs constitute a family of emergent SGQMs. SGQMs are characterised by fully spin-polarized topological transport, featuring unique functionalities of quantum materials as explained above.
The study
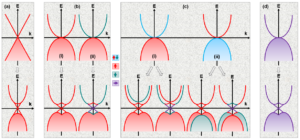
Schematic representation for QAH effect in SGSs. QAH effect in a) Dirac type-I SGSs, b) Dresselhaus type-I SGSs (i) and Rashba type-II SGSs (ii), c) SU(3) SGSs of type-III (i), and type-IV (ii), and d) Rashba type-I SGSs.
The FLEET research team reviewed theoretical and experimental progress in the SGSs and provided a new perspective to uncover underlying physics and the utilisation of SGSs in quantum technologies. This study sheds light on various quantum materials hosting spin gapless behaviour, symmetry constraints leading to spin gapless features, theoretical models characterising SGSs, and device structures and mechanisms for quantum technologies made of SGSs.
Various types of SGSs are described by minimal two-band models characterising Dirac, Dresselhaus, and Rashba Hamiltonian, which explain the underlying physics of the majority of quantum materials. Such a classification of SGSs into Dirac SGSs, Dresselhaus SGSs, and Rashba SGSs allows for the identification of a new class of SGSs known as spin-orbit coupled SGSs. Thus, unlike a conventional wisdom of SGS behaviour in magnetic structures, spin-gapless behaviour can be realised in non-magnetic structures, provided the material exhibits a nontrivial topological feature.
A convergence of topology and magnetism, known as Chern magnetism, makes SGSs ideal candidate materials for realizing the quantum anomalous Hall (QAH) effect. In addition, the QAH effect reported in various other condensed matter systems can also be understood from a perspective of the QAH effect in SGSs. In magnetic SGSs, SOI opens a Chern insulating gap leading to the QAH phase. On the other hand, in spin-orbit coupled SGSs, magnetic exchange interaction opens a Chern insulating gap leading to the QAH phase. Such inherently present Chern magnetism in SGSs could solve a long-awaited problem: achieving the QAH effect at room temperature, explains Dr. Muhammad Nadeem (University of Wollongong), who is a Research Fellow at FLEET.
In this study, yet another classification of SGSs, based on band degeneracy, is introduced. In this context, while twofold degenerate type-I and type-II SGSs are described by SU(2) symmetry, threefold degenerate type-III and type-IV SGSs are referred to as SU(3) SGSs. It extends the idea of spin-gapless behaviour and the associated QAH effect in non-conventional condensed matter systems with multifold band crossings, describing high-spin fermionic quasiparticles beyond Dirac, Weyl, and Majorana fermions that are not permissible in particle physics.
Quantum technologies exploit unusual effects of quantum mechanics and utilise unique properties of quantum materials to engineer quantum devices that have new functionalities and capabilities in computing, communication, and sensing.
Applications in future quantum technologies
The emergent quantum technologies aim to achieve three critical attributes: ultra-fast speed, ultra-high sensitivity, and ultra-low power consumption. However, physical realizations of contemporary quantum technologies are held back because a gap between fundamental science and its implementation is not fully understood yet. The exotic electronic, magnetic, and thermal properties of SGSs render them an ideal platform for developing quantum technologies that can meet the three ‘ultra’ criteria, says Prof Xiaolin Wang (University of Wollongong), who is a Chief Investigator of FLEET. That is, device structures and mechanisms for various quantum technologies making use of SGSs and the associated QAH phase, such as tunnelling junction devices and topological quantum transistors configuring SGSs, promise enhanced functionalities and capabilities.
Spin gapless quantum materials and devices was published in Advanced materials in July 2024 (DOI: 10.1002/adma.202402503)
The Centre for Future Low-Energy Electronics Technologies (FLEET) is a collaboration of over a hundred researchers, seeking to develop ultra-low energy electronics to face the challenge of energy use in computation, which already consumes 8% of global electricity, and is doubling each decade.
Dr. Muhammad Nadeem (University of Wollongong) is a Research Fellow in Prof Xiaolin Wang’s group at UOW node of FLEET, with a research focus on theoretical modelling and numerical simulations for quantum materials and quantum technologies.
Prof Xiaolin Wang leads FLEET’s University of Wollongong node, as well as leading the Enabling Technology team developing the novel and atomically thin materials underpinning FLEET’s search for ultra-low energy electronics, managing synthesis and characterisation of novel 2D materials at the University of Wollongong. Prof Wang is also the Director of Institute of Superconducting and Electronic Materials, UOW.
More information
- Contact Muhammad Nadeem (University of Wollongong) mnadeem@uow.edu.au
- Contact Prof Xiaolin Wang (University of Wollongong) xiaolin@uow.edu.au