Tiny device mimics human vision and memory abilities
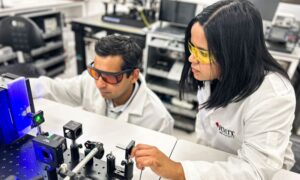
Researchers have created a small device that ‘sees’ and creates memories in a similar way to humans, in a promising step towards one day having applications that can make rapid, complex decisions such as in self-driving cars.
The neuromorphic invention is a single chip enabled by a sensing element, doped indium oxide, that is thousands of times thinner than a human hair and requires no external parts to operate.
RMIT University engineers led the work, with contributions from researchers at Deakin University and the University of Melbourne.
The team’s research demonstrates a working device that captures, processes and stores visual information. With precise engineering of the doped indium oxide, the device mimics a human eye’s ability to capture light, pre-packages and transmits information like an optical nerve, and stores and classifies it in a memory system like the way our brains can.
Collectively, these functions could enable ultra-fast decision making, the team says.
Team leader Professor Sumeet Walia said the new device can perform all necessary functions – sensing, creating and processing information, and retaining memories – rather than rely on external energy-intensive computation, which prevents real-time decision making.
“Performing all of these functions on one small device had proven to be a big challenge until now,” said Walia from the School of Engineering.
“We’ve made real-time decision making a possibility with our invention, because it doesn’t need to process large amounts of irrelevant data and it’s not being slowed down by data transfer to separate processors.”
What did the team achieve and how does the technology work?
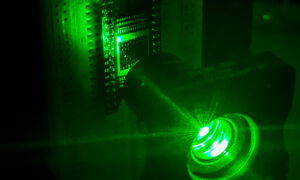
The new device was able to demonstrate an ability to retain information for longer periods of time, compared to previously reported devices, without the need for frequent electrical signals to refresh the memory. This ability significantly reduces energy consumption and enhances the device’s performance.
Their findings and analysis are published in Advanced Functional Materials.
First author and RMIT PhD researcher Aishani Mazumder said the human brain used analog processing, which allowed it to process information quickly and efficiently using minimal energy.
“By contrast, digital processing is energy and carbon intensive, and inhibits rapid information gathering and processing,” she said.
“Neuromorphic vision systems are designed to use similar analog processing to the human brain, which can greatly reduce the amount of energy needed to perform complex visual tasks compared with today’s technologies.”
What are the potential applications?
The team used ultraviolet light as part of their experiments, and are working to expand this technology even further for visible and infrared light – with many possible applications such as bionic vision, autonomous operations in dangerous environments, shelf-life assessments of food and advanced forensics.
“Imagine a self-driving car that can see and recognise objects on the road in the same way that a human driver can or being able to able to rapidly detect and track space junk. This would be possible with neuromorphic vision technology.”
Walia said neuromorphic systems could adapt to new situations over time, becoming more efficient with more experience.
“Traditional computer vision systems – which cannot be miniaturised like neuromorphic technology – are typically programmed with specific rules and can’t adapt as easily,” he said.
“Neuromorphic robots have the potential to run autonomously for long periods, in dangerous situations where workers are exposed to possible cave-ins, explosions and toxic air.”
The human eye has a single retina that captures an entire image, which is then processed by the brain to identify objects, colours and other visual features.
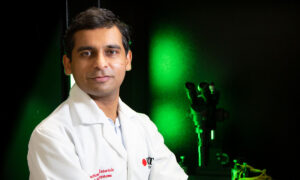
The team’s device mimicked the retina’s capabilities by using single-element image sensors that capture, store and process visual information on one platform, Walia said.
“The human eye is exceptionally adept at responding to changes in the surrounding environment in a faster and much more efficient way than cameras and computers currently can,” he said.
“Taking inspiration from the eye, we have been working for several years on creating a camera that possesses similar abilities, through the process of neuromorphic engineering.”
Story first published: RMIT
‘Long duration persistent photocurrent in 3 nm thin doped indium oxide for integrated light sensing and in-sensor neuromorphic computation’, was published in Advanced Functional Materials in June 2023 (DOI: 10.1002/adfm.202303641)
‘Target identified’: teaching a machine how to identify imperfections in 2D materials
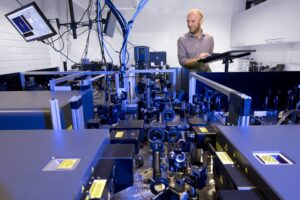
Applying machine learning to automated characterisation of atomically-thin materials
Just as James Cameron’s Terminator-800 was able to discriminate between “clothes, boots, and a motorcycle”, a FLEET study demonstrates potential for machine learning to identify different areas of interest on 2D materials.
The simple, automated optical identification of fundamentally different physical areas on these materials (e.g. areas displaying doping, strain, and electronic disorder) could significantly accelerate the science of atomically-thin materials.
Atomically-thin (or 2D) materials, including metals, semiconductors, insulators, and more-exotic quantum materials such as topological insulators, superconductors and ferromagnets, are studied by scientists around the world seeking to take advantage of their unique properties.
Materials scientists have utilised this monolayer ‘zoo’ to construct next-generation, energy-efficient electronics, batteries, memory cells and photodiodes.
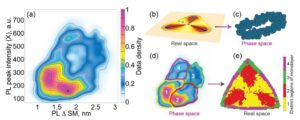
“Without any supervision, machine-learning algorithms were able to discriminate between differently perturbed areas on a 2D semiconducting material,” explains lead author Dr Pavel Kolesnichenko (Swinburne University of Technology). “This can lead to fast, machine-aided characterisation of 2D materials in the future, accelerating application of these materials in future technologies.”
However with the integration of 2D materials into next-generation technologies still involving mostly manual assembly in one-off prototypes, there is still a long way to go to reach industrial-scale production and commercialisation.
Factors that have hindered progress include lack of full control over materials fabrication, the need for experienced oversight of complex characterisation techniques, and the extreme sensitivity of monolayer materials to perturbations, many of which are introduced unintentionally.
Understanding these perturbations is a non-trivial task, as they can have a combined effect and have to be disentangled.
“So many factors can affect opto-electronic properties of 2D materials, including the type of substrate, additional doping, strain, the presence of wrinkles, defects, and environmental molecules – you name it,” says Pavel.
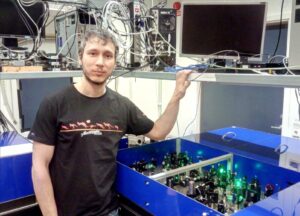
Pavel and Prof Jeff Davis (also at Swinburne) realised that the laborious task of 2D materials characterisation could be accomplished by machines in a rapid and automated manner.
Working with FLEET colleague Prof Michael Fuhrer (Monash University), they applied unsupervised machine-learning algorithms to characterise the semiconducting monolayer tungsten disulphide. The learning algorithms were able to discriminate between the areas on a monolayer flake affected by doping, strain, disorder, and the presence of additional layers.
This is the first time such a systematic disentanglement of these perturbations has been performed.
The team built on previous scientific results in the field including previous work at FLEET, where they disentangled perturbations using correlated photoluminescence and absorption spectra.
In the era of data-driven science and technology, the authors hope that their research will motivate the creation of a large labelled dataset, where labels (such as ‘doping’, or ‘strain’) would be assigned by experienced researchers.
This dataset would be then used to train deep neural networks to characterise 2D materials in a fraction of a second. The researchers believe that their work will help to introduce standards for characterisation of monolayer matter, approaching the moment of large-scale use of low-energy smartphones and computers in the future.
“Disentangling the effects of doping, strain and disorder in monolayer WS2 by optical spectroscopy” was published in 2D Materials in January 2020. (DOI: 10.1088/2053-1583/ab626a)
Superfluids provide new insight into turbulence
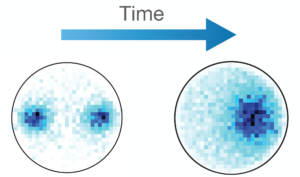
University of Queensland researchers from FLEET and a partner Centre of Excellence experimentally validated a decades’ old vortex theory, with implications for technological applications of superfluid, such as precision sensing.
Eddies in the quantum state known as a superfluid merge to form large vortices, analogous to how cyclones form in the turbulent atmosphere.
Lead author and theorist FLEET Research Fellow Dr Matt Reeves says the team’s results provide experimental validation of the 70-year-old Onsager theory – a model for two-dimensional vortex equilibrium by Nobel Laureate Lars Onsager.
“Large, long-lived vortices like cyclones or Jupiter’s Great Red Spot often form out of turbulent fluid flows, such as the atmospheres of planets,” says Matt.
Onsager’s model explains the existence of these structures, but prior to this 2022 collaborative study with the Australian Research Council Centre of Excellence for Engineered Quantum Systems (EQUS), experiments have tended to conflict with the predictions.
A key complication is that most fluids are viscous, meaning they resist flow. Superfluids, which have no viscosity, are therefore ideal candidates to realise Onsager’s model.
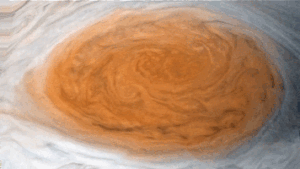
Cyclones, such as Jupiter’s Great Red Spot, are ‘constrained’ in the third dimension (up and down), and thus operate as 2D vortices.
The team studied the behaviour of vortices in a Bose-Einstein condensate, a type of superfluid produced by cooling a gas of rubidium atoms to extremely cold temperatures.
“We created a thin disc of the superfluid and then used lasers to inject vortices at carefully specified locations,” says EQUS researcher Dr Tyler Neely, who led the experiments.
The vortices mixed rapidly, merging into a single large cluster in only a few seconds, much like a large cyclone forming from the turbulent atmosphere.
However, the most exciting thing was the remarkable agreement between theory and experiment – the theory predicted the shape of the final giant vortex structures in the superfluid exceptionally well.
This work answers some of the key outstanding questions from previous work by the team on vortex clusters, which was published in 2019 in Science.
This article first published by ARC Centre of Excellence for Engineered Quantum Systems
Turbulent Relaxation to Equilibrium in a Two-Dimensional Quantum Vortex Gas was published in Physical Review X in February 2022 (DOI: 10.1103/PhysRevX.12.011031.
Advancing quantum interaction understanding in 2D semiconductors
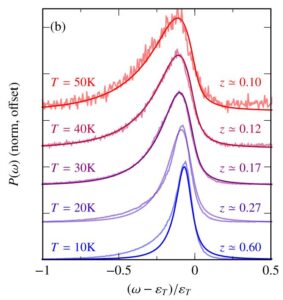
Unlocking fresh insights into the behaviour of quantum impurities within materials
An international theoretical study led by Monash University researchers introduced a novel approach known as ‘quantum virial expansion’ – a powerful tool to uncover the complex quantum interactions in 2D semiconductors.
This breakthrough holds potential to reshape our understanding of complex quantum systems and unlock exciting future applications utilising novel 2D materials.
The study of ‘quantum impurities’ has far-reaching applications across physics in systems as diverse as electrons in a crystal lattice and protons in neutron stars. These impurities can collectively form new quasi-particles with modified properties, essentially behaving as free particles.
The new technique shows remarkably good agreement with experimental results, essentially perfect at high temperature with small discrepancies at lower temperatures.
Although a straightforward many-body problem to state, quantum impurity problems are difficult to solve.
“The challenge lies in accurately describing the modified properties of the new quasi-particles,” says Dr Brendan Mulkerin (Monash), who led the collaboration with researchers in Spain.
The study offers a novel perspective on exciton-polarons as impurities in 2D materials – bound electron-hole pairs immersed in a fermionic medium.
As a solution to the problem, the Monash team introduced the ‘quantum virial expansion’ (QVE), a powerful method that has long been indispensable in ultra-cold quantum gases.
In this case, integrating QVE into the study of quantum impurities meant that only the interactions between pairs of quantum particles needed to be taken into account (i.e. rather than interactions between large numbers of particles). The resulting, solvable model sheds new light on the interplay between impurities and their surroundings in 2D semiconductors.
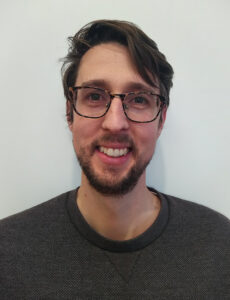
The new approach is remarkably effective at relatively high temperatures (e.g. in a semiconductor anything above a few degrees Kelvin) and low doping (where the electrons’ thermal wavelength is smaller than their interparticle spacing), leading to a ‘perturbatively’ exact theory (referring to a quantum system being perturbed from a simple, solvable limit).
“One of the most intriguing aspects of this research is its potential to unify different theoretical models, with the ongoing debate surrounding the appropriate model for explaining the optical response of 2D semiconductors being resolved through the quantum virial expansion,” says corresponding author A/Prof Jesper Levinsen (also at Monash).
The quantum virial expansion is expected to have a broad impact, extending its applications to various systems beyond 2D semiconductors.
“Understanding quantum impurity physics will continue to reveal insights and unlock novel properties and new possibilities for understanding, harnessing, and controlling quantum interactions,” says corresponding author Prof Meera Parish (Monash).
“Exact quantum virial expansion for the optical response of doped two-dimensional semiconductors” was published in Physical Review Letters in September 2023 (DOI: 10.1103/PhysRevLett.131.106901)